Transform your ideas into professional white papers and business plans in minutes (Get started for free)
Scientific Parallels in Rivers Solomon's HSS Matilda A Deep Dive into the Physics of Generation Ships
Scientific Parallels in Rivers Solomon's HSS Matilda A Deep Dive into the Physics of Generation Ships - Generation Ship Physics Behind HSS Matilda Propulsion System
Delving into the physics powering the HSS Matilda, Solomon's fictional generation ship, reveals the core engineering challenges inherent in interstellar travel. Sustaining a crew across generations necessitates not only the provision of resources for survival but also the creation of an environment that mimics Earth's gravity. This likely involves incorporating rotating structures, a common concept in generation ship designs, to provide a semblance of normalcy for the inhabitants. Moreover, the ship's propulsion system, potentially powered by fusion, highlights the need for efficient and sustainable energy sources over centuries of travel. This long-duration journey inevitably raises questions about the sheer energy demands and how those might impact the ship's structure and the living conditions on board. The technological hurdles are formidable, especially considering the inherent psychological and biological stresses on a multi-generational crew. This exploration of advanced engineering in a fictional context ultimately underscores the complex interplay between humanity's ambitions for space and the formidable biological and environmental challenges they'll face if venturing into the unknown.
The HSS Matilda's proposed propulsion system hinges on a speculative fusion reactor, capitalizing on the immense energy liberated when hydrogen isotopes fuse. If realized, this process would offer practically inexhaustible power, akin to the output of stars themselves. This power is then manipulated by a theoretical system of sophisticated electromagnetic fields. This design minimizes mass loss during propulsion, a critical consideration for ensuring the vessel's long-term energy efficiency and sustainability.
Some researchers speculate that the Matilda's form is optimized for plasma flow from the propulsion system. This is akin to the streamlined bodies of fish or birds, aiming to decrease drag and improve the thrust-to-weight ratio.
The hypothetical Matilda propulsion system likely relies on superconductor materials maintained at extremely low temperatures. This, in theory, could vastly improve the efficiency of electrical systems within the harsh environment of space, allowing for the conveyance of substantial currents with minimal energy loss.
Navigating interstellar space with the Matilda would probably involve a combination of artificial intelligence and intricate quantum algorithms. These advanced tools would map the most efficient routes through the cosmos, a testament to the development of complex physics simulations.
Relativity plays a crucial role in charting the Matilda’s trajectory and its operational schedule. The ship's tremendous speeds would result in notable time dilation, a consequence where the passage of time onboard differs significantly from time on Earth. This effect must be carefully accounted for when managing crew aging and overall mission planning.
The ship's design, it is theorized, relies on novel materials engineered to endure the harshness of space travel. Such materials are crucial to withstand impacts by micrometeorites, exposure to high radiation levels and the extremes of space's thermal fluctuations.
The HSS Matilda’s life support systems are built around a closed-loop water recycling technique. This strategy minimizes the need for external resources, mechanically imitating natural biogeochemical cycles. It creates a complex, but self-sustaining, ecosystem within the vessel.
The enormous energy requirements of the ship's propulsion and life support systems necessitate a creative approach to energy acquisition. It has been proposed that arrays of solar-collecting satellites could be deployed to harness solar energy as the ship journeys across the vast distances of space.
One theory underpinning the ship's design proposes the use of gravitational assists from planetary bodies to accelerate the Matilda. Employing Newton's laws, the ship could potentially achieve greater velocities without an excessive energy expenditure. This echoes strategies employed in current space exploration programs, but scaled up to an interstella context. While fascinating, the viability and engineering challenges of each aspect of such a vessel remain open to discussion and refinement.
Scientific Parallels in Rivers Solomon's HSS Matilda A Deep Dive into the Physics of Generation Ships - Artificial Gravity Methods and Centrifugal Force in Deep Space Travel
Artificial gravity (AG) becomes crucial for ensuring the health and well-being of humans during extended voyages in space, especially within the context of generation ships like the HSS Matilda. Creating artificial gravity involves leveraging centrifugal force generated by rotation to mimic the effects of Earth's gravity. This is seen as a countermeasure to the adverse physiological consequences of extended periods of weightlessness. The design of the spacecraft, including the rate of rotation and the distance from the axis of rotation, are essential factors in producing effective artificial gravity. However, significant hurdles remain, including the complex biological and mechanical responses of the human body to AG, especially the variation in gravitational force experienced by different body parts. While artificial gravity frequently appears in science fiction, the actual engineering challenges and potential applications have received relatively little attention, emphasizing both the exciting possibilities and the considerable hurdles associated with interstellar travel. The success of creating functional artificial gravity goes beyond simply ensuring the survival of spacefarers; it's a critical step towards enabling a sustainable human presence in the vast and challenging environment of deep space.
Generating artificial gravity (AG) using centrifugal force, essentially mimicking the inertial force of gravity through rotation, is a key concept in spacecraft design, particularly for long-duration missions in deep space. Research over the last 15 years has underscored how crucial AG is to counteracting the negative impacts of extended periods in microgravity on human physiology. The challenge lies in finely tuning the rotational speed of the craft and the distance from the axis of rotation to create a level of AG suitable for astronauts.
This search for the "right" level of AG highlights a major hurdle—understanding how the human body adapts and responds to AG. There's ongoing debate about the practical limits of rotation rates and the radius of the rotation itself. One immediate challenge is the difference in experienced gravity across the body in a rotating frame. Since the head is closer to the axis of rotation, it will experience less gravity than the feet.
The good news is that increasing the rotation rate (RPM) reduces the radius required for effective AG, making designs for spacecraft more practical. However, we've seen this idea in science fiction far more than in realistic engineering. The limited engineering focus on AG is largely due to the significant resources required and the complexity of studying the human body's response to long-term artificial gravity exposure.
The fact that astronauts in orbit experience weightlessness relative to their spacecraft further emphasizes the need for efficient AG systems on longer missions. Common design ideas for these systems revolve around large rotating toroids (doughnut shapes) to best accommodate human needs in space.
This is where things get complicated. It's easy to imagine a spinning wheel in space, but the reality of engineering such a system for a generations-long journey is full of unknowns. The engineering challenges come from allocating the necessary resources and the potential for unexpected physiological effects in humans living under constant artificial gravity. Will there be side-effects? Will people get used to a lower 'gravity' than Earth's? How will we design systems with constantly changing rates of rotation to account for acceleration and deceleration phases of the journey? These are crucial questions that must be answered before we can even think of sending humans on generation ship missions.
Scientific Parallels in Rivers Solomon's HSS Matilda A Deep Dive into the Physics of Generation Ships - Life Support Systems and Resource Management During 325 Year Journey
The success of the HSS Matilda's 325-year journey hinges on its ability to effectively manage life support and resources. The ship, much like Earth's natural cycles, relies heavily on closed-loop systems. These systems, employing advanced recycling techniques, create a self-contained environment to minimize the need for external resources—an absolute necessity given the immense distances of interstellar travel. Maintaining a balanced and sustainable ecosystem within the ship becomes a primary challenge. This requires intricate planning and management of food production, water recycling, and waste processing. Generating a continuous food supply for multiple generations is crucial, demanding a detailed approach to agriculture within a confined space. Furthermore, the ship's massive energy needs must be addressed through a variety of methods. Harvesting solar energy with vast arrays, or perhaps exploring other less explored methods, becomes an integral part of the ship's resource management. These complex systems must also demonstrate flexibility, adapting to the shifting needs of the diverse population aboard while keeping the Matilda's environment balanced and healthy throughout the lengthy voyage. Adaptability and resilience become key in ensuring the longevity of the entire mission.
Sustaining human life across a 325-year journey in a generation ship like the HSS Matilda demands incredibly complex life support systems. These systems must operate in a closed loop, essentially creating miniature, self-sufficient ecosystems. We're talking about a level of engineering that requires an intimate understanding of biochemistry and systems engineering. The complexity is staggering, but essential for long-term survival.
Water, as always, is a cornerstone. In space, relying on traditional methods simply won't work. We'd need advanced technologies like membrane filtration and sophisticated condensation systems to recycle and purify it. It's a necessity since minimizing the ship's freshwater footprint is crucial.
Oxygen production for a 325-year voyage poses a challenge, too. Mimicking Earth's natural processes, we'd likely see sophisticated photobiological systems using algae or plants to convert carbon dioxide into oxygen. This is an ingenious double-duty system since it would produce both breathable air and food resources—a smart solution to resource management.
Resource management within a generation ship needs careful consideration. I imagine a highly-developed approach using AI-powered predictive algorithms. This would help optimize inventory and automate maintenance schedules, ensuring critical supplies are readily available without excessive waste.
One area that often gets overlooked is radiation. Deep space travel exposes the crew and all the technology to intense radiation over time. Developing shielding mechanisms using water or innovative new materials is crucial to mitigate this risk, safeguarding the crew and the ship's systems.
Energy provision is multifaceted, likely using a tiered system. Solar panels would provide auxiliary power but a robust, perhaps nuclear or fusion, reactor would be the primary power source. Diversifying the energy supply makes the ship less vulnerable during unforeseen journey phases.
Thermal management is also critical. Maintaining the appropriate temperature in a sealed environment necessitates sophisticated control systems. We'd need to blend passive and active thermal control techniques to ensure comfortable and functional living conditions.
Just as vital as the physical environment is the psychological well-being of the crew. The constant isolation and the unique stresses of this kind of journey could take a toll on mental health. Designers need to thoughtfully include social spaces, simulated natural environments, and access to natural light—all part of fostering a positive crew environment.
We may also see a concept known as 'food forests', a method of agriculture in which a dense ecosystem maximizes food production in a small area. Beyond just the nutritional aspect, this idea has psychological benefits by bringing a piece of Earth's biodiversity into the ship, which could benefit crew morale and potentially address psychological isolation.
Last but not least, we've got the maintenance challenge. Replacing parts and upgrading aging life support systems over 325 years presents a monumental challenge. We need to embrace cutting-edge materials science and nanotechnology to create self-repairing components or at least allow for robust repairs with minimal external resources. The idea of relying on resupply simply doesn't work over such huge distances and time scales.
Scientific Parallels in Rivers Solomon's HSS Matilda A Deep Dive into the Physics of Generation Ships - Temperature Control and Heat Distribution Across Ship Decks
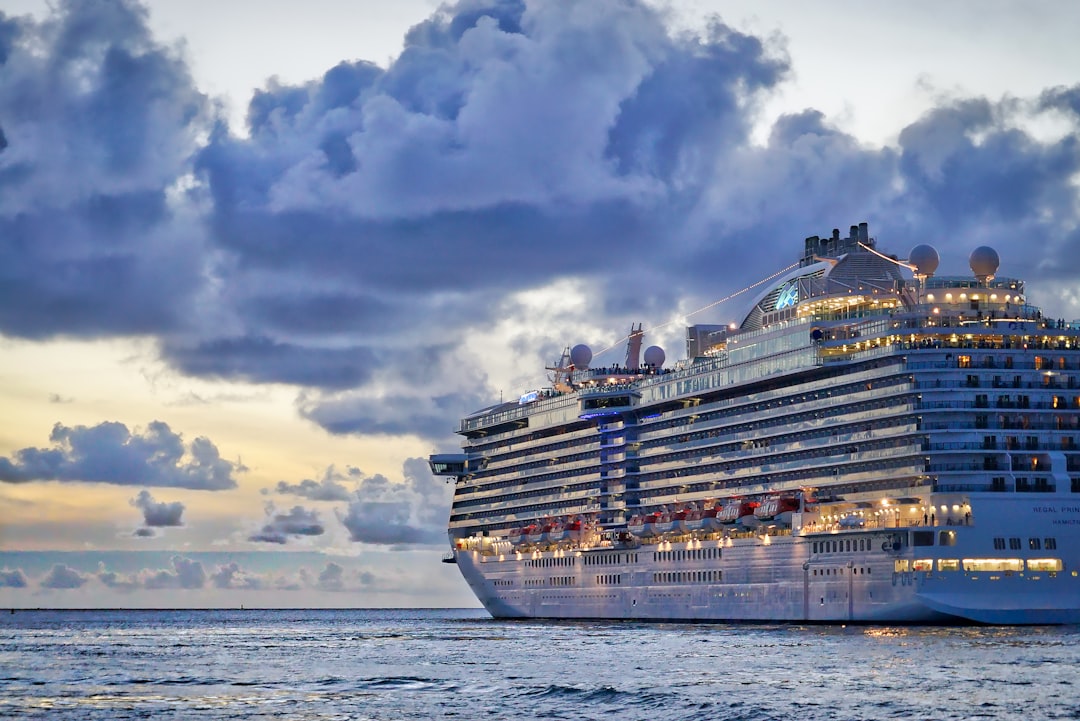
Maintaining consistent temperatures and managing heat distribution across the multiple decks of a generation ship like the HSS Matilda poses significant engineering challenges. The ship's complex structure demands a sophisticated thermal management system to provide comfortable living conditions for the crew and safeguard the integrity of essential systems. Modeling the ship's thermal behavior using methods like a Volume Element Model (VEM) is crucial. Such models can predict and analyze heat flow, potentially identifying hot spots that could impact crew well-being or compromise operational integrity. Thinking about how the ocean's capacity to absorb excess heat relates to atmospheric conditions on Earth can inform understanding of the unique environment within the ship's closed ecosystem. The need to distribute heat evenly is therefore not just an engineering issue, but a key component of maintaining a stable and healthy environment in the challenging conditions of deep space. It is a factor that influences both crew safety and mission success.
### Temperature Control and Heat Distribution Across Ship Decks
Thinking about the challenges of a generation ship like the HSS Matilda, maintaining comfortable and functional temperatures across multiple decks for centuries presents a huge engineering challenge.
One big consideration is **thermal stratification**, where heat naturally rises. This can create significant temperature differences between the upper and lower decks. We'd need advanced ventilation systems to effectively circulate air and create a more even temperature distribution, both for comfort and to prevent damage to equipment.
The choice of materials for building the ship has a significant impact on temperature control too. Materials with high thermal conductivity could be useful in places like the propulsion system, where rapid heat transfer is desirable. However, in the living areas, it's probably better to use materials that don't transfer heat quickly. This minimizes energy use and helps to maintain a stable internal temperature.
**Phase change materials (PCMs)** could be a neat trick for controlling temperature swings. These substances absorb or release heat during their phase transitions, like melting or freezing. They could act as thermal buffers, helping to smooth out variations in energy demand or external temperature changes, such as passing through radiation zones.
Thinking beyond passive approaches, we can use **active thermal control systems**, like thermoelectric devices. These systems could efficiently manage temperatures in various parts of the ship and even potentially convert waste heat into usable energy. This would help maximize efficiency.
**Heat exchangers** could play a critical role in maintaining optimal temperatures. Strategically placed, they could move heat from warmer areas to colder areas, helping to balance things out. This would be especially important for maintaining sensitive life support systems and crew comfort.
**Thermal insulation** is also essential. Using appropriate insulating materials in walls and other surfaces could drastically cut down on heat loss and make it easier to keep the ship's interior at a stable temperature. This would be crucial for long-term habitability.
Beyond the purely engineering side, temperature control has a strong impact on crew **psychological well-being**. Studies have shown that temperature variations can affect mood and stress levels. Maintaining consistent, comfortable temperatures becomes even more vital for the success of a generation ship mission, considering the already demanding environment and the potential for psychological isolation on a centuries-long voyage.
We might consider **localized heating systems** like radiant panels to control temperature in specific areas only. This would be more energy-efficient than heating the entire ship, and would allow for more individual preferences.
**Dynamic feedback systems** with sensor networks could monitor temperature changes throughout the ship in real-time. These systems could make adjustments to heating and cooling as needed, adapting to changing occupancy levels and conditions.
Finally, **heat recycling strategies** could capture wasted heat from the ship's engines or life support systems and reuse it. This would boost efficiency and reduce the strain on the overall thermal management systems.
It's clear that managing the temperature on a generation ship is a complex issue, intertwining with material science, engineering, psychology, and resource management. Tackling these challenges head-on is critical to ensuring a successful journey.
Scientific Parallels in Rivers Solomon's HSS Matilda A Deep Dive into the Physics of Generation Ships - Space Radiation Effects on Multi Generational Ship Communities
The harsh environment of space, particularly the constant exposure to radiation, poses a major threat to the health and long-term survival of multi-generational ship communities like those imagined in the HSS Matilda. Cosmic radiation, a persistent danger in deep space, can cause a variety of harmful effects on living organisms, including DNA damage, an increased risk of cancer, and potentially genetic mutations that accumulate across generations. These risks highlight the crucial need for ship designers to prioritize radiation shielding and resource management strategies to minimize exposure. Moreover, the closed nature of these environments means that genetic diversity within the population can be affected by radiation-induced mutations. This makes monitoring genetic health and mitigating the risks of inbreeding critical for sustaining a viable community over centuries. Understanding and addressing these radiation-related challenges is crucial for ensuring the long-term health and survival of a generation ship's inhabitants and the success of interstellar voyages.
The long-term effects of space radiation on multi-generational ship communities are a major hurdle for interstellar travel. The cumulative dose of radiation exposure across generations could lead to unexpected genetic changes, influencing traits such as physiology, growth, and disease susceptibility. We need to better understand this area, particularly given the different types of radiation in space.
Galactic cosmic rays (GCRs) and solar particle events (SPEs) pose different challenges. GCRs, primarily high-energy protons and heavier ions, are especially problematic since they can penetrate traditional shielding more easily. This means we need to develop new approaches beyond traditional methods like lead shielding, which can be too heavy for spacecraft. Ideas like using water tanks, polyethylene, or specialized composites might be more practical.
The effects of radiation extend beyond physical health. Psychological stress due to prolonged isolation and the inherent uncertainty of radiation levels could exacerbate existing disorders in crew members. This means living quarters and social spaces must be designed with the psychological aspects of radiation in mind.
To proactively address radiation exposure, we need to incorporate robust radiation monitoring systems into ship designs. This could include advanced isotopic or real-time monitoring tools to track cumulative doses for individual crew members. We can then adjust living conditions, work hours, and other factors to mitigate exposure.
Looking to nature might provide insights. Organisms like tardigrades have remarkably evolved to withstand extreme radiation environments. Understanding their cellular mechanisms could inform future human engineering to enhance our resilience to radiation stress.
The effects of radiation on reproduction are another serious consideration. Potential impacts on fertility or fetal development during extended missions are a major ethical consideration.
However, the paradox is that space radiation might push us to develop breakthroughs in gene therapy and regenerative medicine. Understanding how cells repair damage from radiation could lead to advancements for those on Earth affected by radiation exposure.
The delicate balance of a generation ship's ecosystem could be disrupted by radiation. Increased radiation levels could upset the biogeochemical cycles, potentially harming the essential microflora needed for oxygen and waste management. This directly impacts the ship's life support system.
Lastly, radiation doesn't just pose biological risks, it also creates thermal stresses on spacecraft materials. This can lead to degradation over time. We need to carefully consider the interplay of radiation and thermal management during ship design to ensure structural integrity across generations. There's still a lot of research needed to make this a reality, but the rewards are potentially vast for both interstellar travel and advancing medical care on Earth.
Scientific Parallels in Rivers Solomon's HSS Matilda A Deep Dive into the Physics of Generation Ships - Power Generation and Energy Conservation in Deep Space Vessels
Deep space vessels, like the imagined HSS Matilda, require robust and adaptable power systems to sustain long-duration journeys. The extreme conditions of space necessitate the development of power generation methods beyond those used on Earth. Traditionally, solar panels have been a cornerstone of space exploration, but their effectiveness can be limited in deep space where sunlight is scarce. Consequently, generation ships might rely on advanced technologies, such as nuclear electric propulsion (NEP) or radioisotope power systems (RPS), offering a stable energy supply regardless of solar exposure. These systems could power essential functions from life support to propulsion. Furthermore, the field of energy generation is continually evolving, with ongoing research exploring methods to leverage hybrid power sources and even harness the cold of space itself for energy. Finding diverse and efficient energy solutions is crucial not just for the practical needs of a ship, but also for the health and well-being of its inhabitants during a generations-long journey across the vast interstellar expanse. This diverse approach to energy is essential for a sustainable mission and the success of multi-generational deep space travel.
Deep space travel demands a comprehensive approach to power generation, storage, and distribution, particularly for the extended durations involved in interstellar voyages, and for both crewed and uncrewed missions. Developing advanced energy sources with high specific power and durability is a priority, given the harsh environmental conditions encountered during deep space exploration.
Nuclear electric propulsion (NEP) has shown promise for this very reason, its ability to provide substantial power compared to solar or radioisotope systems making it a theoretical frontrunner for future exploration. Radioisotope power systems (RPS), despite being used historically for more localized tasks, continue to be valuable due to their reliable power output even in environments lacking sunlight.
There's a growing focus on hybrid power and propulsion systems, which have the potential to improve both the efficiency and reliability of power generation and storage. This has relevance beyond space travel; insights gained could potentially improve both marine and terrestrial energy technologies. We've already seen this in advancements to solar panels, but there's always room for improvement. For instance, the concept of using Earth's nocturnal heat for energy generation has relevance to space travel, where finding and using alternative energy sources will be critical for sustained missions.
We might glean further useful concepts from marine technologies, such as energy harvesting from ocean waves. The synergies between space exploration and deep-sea research are increasingly evident, particularly in areas such as remote sensing and monitoring. Analyzing the effectiveness of deep-sea hydrothermal energy generation systems via multiphysics cosimulation offers another area for valuable parallels in optimizing power systems for long-duration missions in space.
Researchers are exploring even more novel concepts, such as the possibility of harvesting the deep cold of space for energy generation. While still in the conceptual stages, it suggests a path towards potentially diverse energy solutions for future explorations. This underscores a need to consider the cold side of space as a possible source of energy, just as we've looked at the hot. Each of these challenges is complex, and finding a way to combine many sources of power generation, including nuclear, solar, and fusion power, will require novel solutions. Finding those solutions is going to take time and be a difficult and intricate process.
Transform your ideas into professional white papers and business plans in minutes (Get started for free)
More Posts from specswriter.com: