7 Critical Engineering Challenges in Writing Active Transportation Plans for Urban Areas
7 Critical Engineering Challenges in Writing Active Transportation Plans for Urban Areas - Integration of Micromobility Networks with Traditional Transit Routes in Dense Urban Cores
Connecting micromobility networks—like bike and scooter share programs—with established public transportation systems within crowded city centers offers a powerful way to improve urban mobility. Integrating these systems requires a proactive approach within urban planning, ensuring spaces are designed to welcome micromobility and facilitate smooth transitions between them and buses, trains, and other transit options. This is vital for overcoming the well-known challenge of "first and last mile" connectivity, which often hinders wider transit adoption.
However, achieving this interconnectedness is often hampered by a lack of collaboration between private micromobility companies and public transit agencies. This can result in fragmented services and inefficient operations. Therefore, developing clear standards and recommendations for creating infrastructure that fosters this integration is essential. This kind of proactive, well-planned approach is crucial to maximizing the benefits of micromobility for all residents, specifically those in underserved communities, by improving access to jobs, education, healthcare, and other vital aspects of urban life. In essence, by thoughtfully weaving micromobility into the fabric of the city's transportation system, we can strive towards a more unified, efficient, and equitable transportation ecosystem for everyone.
Weaving micromobility into the fabric of existing public transit networks within densely packed urban centers presents a fascinating challenge. While micromobility services like bike-sharing and e-scooters are gaining traction as a means to address diverse transportation needs across various regions, integrating them seamlessly with traditional transit remains an ongoing experiment. The idea is compelling – these systems could address the "first-mile/last-mile" problem, thereby making public transit more appealing and expanding its reach.
This potential for increased transit ridership is particularly attractive in areas where access to jobs, education, and healthcare is limited, potentially improving equity in mobility. We also see the promise of extending the "catchment area" of transit stops, essentially enlarging the geographical sphere of influence of each station.
How exactly can we achieve this integration? One approach is through physical integration—designing infrastructure to facilitate easy transfers—and the other is through payment systems that offer convenient multimodal ticketing options. Current research predominantly relies on analyzing trip data to glean insights into the travel patterns of those who use micromobility options in conjunction with other modes.
However, the landscape is complex. For instance, many privately-run bike and scooter companies operate independently of public transit agencies, sometimes even fostering competition rather than productive partnerships. This lack of collaboration can be a roadblock to efficient, integrated systems. We therefore need guidelines and best practices that help us design infrastructure that efficiently supports the combined use of micromobility and public transportation.
This complex integration is vital if we're to develop holistic transportation solutions. Some cities, like Seattle, are still grappling with implementation. Without proper consideration and execution, the benefits of a micromobility-enhanced public transit network might not be fully realized. The future of urban mobility may depend on figuring out how these elements can work together harmoniously.
7 Critical Engineering Challenges in Writing Active Transportation Plans for Urban Areas - Capacity Analysis Methods for Mixed Use Paths During Peak Hours
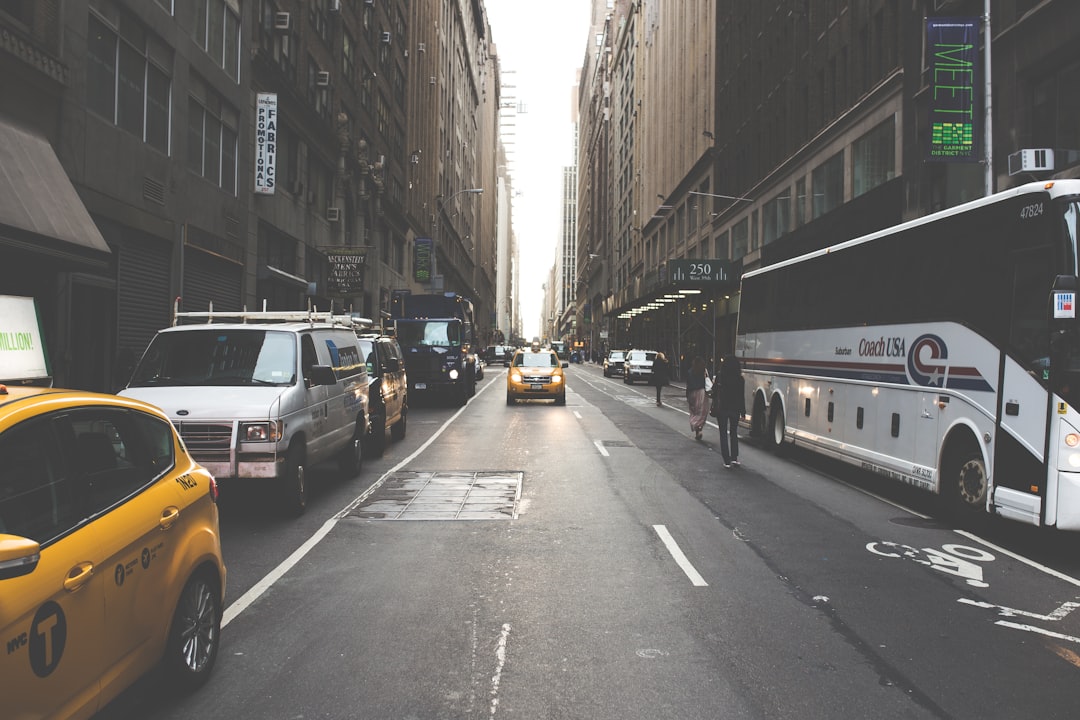
Understanding how many people can safely and comfortably use shared paths for walking and cycling during peak hours is essential for managing urban mobility. This requires sophisticated analysis methods that consider both predictable and unpredictable factors. Analyzing how these paths function dynamically, considering interactions between people on foot, bicycles, and possibly other modes, is important. This involves applying established frameworks, such as the Highway Capacity Manual, which offer ways to measure traffic flow and quantify the impact of peak usage times. To properly plan for these paths, planners need to understand how the volume of people changes throughout the day and design paths to accommodate everyone.
This analysis ideally incorporates mathematical modeling, which can help determine the maximum capacity for each section of the path. This helps guide decisions about design features that maximize safety and capacity. By carefully considering the principles of capacity analysis within active transportation plans, engineers and planners can improve the effectiveness and equity of urban mobility systems, contributing to a more sustainable, and just urban environment.
1. Mixed-use paths, designed for pedestrians, cyclists, and sometimes other users, present unique challenges for capacity analysis due to the interactions between different user types. Understanding these interactions and their impact on path usage is vital for accurate assessments.
2. Peak hour analysis reveals how user behavior significantly shifts between weekdays and weekends. Commuter patterns dominate during the week, while leisure activities are more prevalent on weekends. This variability necessitates adaptive strategies to accommodate these different usage patterns.
3. Path width plays a crucial role in determining capacity. Wider paths generally lead to higher speeds and increased capacity, though this relationship isn't linear. Striking a balance between maximizing capacity and ensuring comfortable and safe conditions within tighter sections is important.
4. Environmental factors like weather conditions and daylight hours can have a dramatic impact on peak hour usage. Rain, temperature changes, and seasonal variations not only affect user comfort but also alter the number of users and their speeds. This can significantly impact capacity calculations.
5. Amenities along the paths, while improving the user experience, can disrupt flow and potentially decrease overall capacity. Benches, water fountains, and rest stops all require space, which needs to be thoughtfully integrated into the path design to avoid bottlenecks.
6. Similar to vehicular traffic, pedestrian and cyclist interactions can lead to "bunching" during peak times. This phenomenon reduces individual speeds and lowers overall path capacity, prompting a need for design solutions that aim to minimize congestion.
7. Research indicates that injury rates on mixed-use paths rise during peak periods, highlighting a crucial need for safety analyses alongside capacity assessments. A careful balance is needed to promote both user safety and efficient flow.
8. The relationship between path surface material and capacity is noteworthy. Smoother surfaces generally allow for higher speeds, leading to increased capacity. This suggests that material selection should consider not just cost but also the needs and expected volumes of the intended user groups.
9. Capacity analysis must account for the fact that new users often have a learning curve, especially during peak periods. These users might be slower or less confident, affecting the overall flow. Path designs should consider this variability in user experience and skill.
10. There's a notable gap between the theoretical calculations often used to determine performance metrics and real-world observations of mixed-use path capacity. This discrepancy points to the need for more empirical data collection and analysis to create more effective design and planning strategies.
7 Critical Engineering Challenges in Writing Active Transportation Plans for Urban Areas - Managing Grade Separation Requirements at Major Urban Intersections
Integrating grade separation into major urban intersections is a crucial aspect of improving urban mobility and safety. Grade separations, including overpasses and underpasses, are designed to eliminate points of conflict between vehicles, pedestrians, and sometimes even rail traffic. This separation helps to enhance traffic flow and reduce the risk of accidents at intersections. However, effectively implementing these projects requires careful consideration of numerous factors.
The design process for grade separations must take into account both structural and geometric elements that are unique to each intersection. It is important to recognize that these features will impact the surrounding streetscape and public spaces. Equally important is that the plans for grade separations must also prioritize pedestrian and cyclist access. We cannot allow urban planning focused on vehicles to disregard the needs of pedestrians and cyclists. It’s essential to integrate walkability into these projects and ensure seamless connections to existing pedestrian infrastructure.
Implementing grade separations can lead to increased intersection capacity and smoother traffic flow, particularly in areas with high traffic volumes. However, achieving this objective requires considering various factors. Decisions regarding the placement of these structures, their design, and connections to other transportation modes significantly affect overall urban mobility and the user experience. Without a thoughtful and inclusive approach, these projects risk simply shifting traffic challenges rather than resolving them.
1. **Designing for Diverse Scenarios:** Grade separations at urban intersections often necessitate intricate design processes. Factors like traffic volume, land use, and even local customs all come into play, making it challenging to create uniform solutions. What works well in one situation might be unsuitable in another, seemingly similar, setting.
2. **Managing Variable Loads:** Different intersection types handle vastly different levels of vehicular traffic. This variability requires sophisticated structural engineering to ensure grade separations don't fail prematurely due to stress and fatigue, especially in areas with heavy traffic.
3. **Accounting for Seismic Activity:** In areas with a history of earthquakes, grade separations have to meet rigorous seismic design standards. This involves complex calculations to ensure stability during seismic events, which can significantly increase both project costs and the time it takes to complete them.
4. **Addressing Elevation Changes:** Even minor changes in elevation related to a grade separation project can have a profound impact on drainage systems. Engineers need innovative approaches to prevent flooding and water pooling in lower areas, which can add complexity to the design process.
5. **Balancing Safety and Flow:** Finding the right balance between traffic flow and safety in grade-separated intersections is a constant challenge. While elevated crossings may improve vehicle speeds, they can also create blind spots that pose hazards for pedestrians and cyclists. Comprehensive planning is crucial to mitigate these risks.
6. **Designing for All Users:** Grade separation doesn't just affect cars—it significantly impacts pedestrian access as well. Designing elements like escalators and elevators can improve usability, especially for people with mobility limitations. This highlights the need for inclusivity when developing these designs.
7. **The Challenge of Phased Construction:** Building grade separations in urban environments is often complex. Phased construction schedules are common, meaning projects can take longer and disrupt surrounding communities. Carefully managing these stages is important to minimize disruptions.
8. **Land Acquisition Hurdles:** Securing the land necessary for a grade separation project can be a major obstacle, especially in dense urban areas where space is at a premium. This can lead to lengthy legal processes, bureaucratic hurdles, and project delays, potentially driving up costs as well.
9. **Material Choices and Costs:** The materials used in a grade separation structure—for bridges or tunnels, for example—have a major impact on the overall budget and future maintenance. Engineers must carefully consider the trade-offs between initial cost and the structure's longevity, looking for a balance that optimizes both.
10. **Integration with Existing Systems:** Combining new grade separations with older road systems and infrastructure can lead to unexpected issues. Pre-existing elements might need to be retrofitted or altered, leading to complications that can affect the efficiency and overall scope of the project.
7 Critical Engineering Challenges in Writing Active Transportation Plans for Urban Areas - Traffic Signal Optimization for Pedestrian and Cyclist Priority Zones
Optimizing traffic signals is vital for creating urban environments that prioritize pedestrians and cyclists. Traditional signal timing often emphasizes minimizing vehicle delays, overlooking the often-longer delays faced by pedestrians and cyclists. This can lead to inequitable experiences where those on foot or bicycle endure significantly longer wait times. As cities integrate new technologies, like connected and automated vehicles, optimizing traffic signals becomes increasingly complex. The opportunity to optimize signal timings to benefit all modes of transport becomes more tangible. This includes the possibility of incorporating more adaptive methods like deep reinforcement learning, which can more effectively manage traffic flow at intersections. The challenge is to develop comprehensive solutions that balance the needs of all road users, considering factors like safety and equity while developing a more vibrant, pedestrian-friendly urban experience.
Optimizing traffic signals to prioritize pedestrians and cyclists in urban environments is a growing area of focus. While traditional signal timing often prioritizes minimizing vehicle delays, the needs of pedestrians and cyclists are frequently overlooked, leading to longer average wait times for vulnerable road users. In some cases, vehicle delays might be as low as 20 seconds at intersections, while pedestrian delays can stretch up to 80 seconds, highlighting a clear imbalance in current practices.
The rise of adaptive signal control technology (ASCT) is offering a promising avenue for change. By leveraging sensor and camera data, ASCT allows real-time adjustments to signal timing, potentially reducing delays and enhancing safety. Furthermore, the incorporation of dedicated pedestrian phases at intersections has shown considerable promise in reducing pedestrian-vehicle conflicts, with studies showing up to a 40% decrease in related collisions. Similarly, dedicated signal phases for cyclists can improve both their safety and their compliance with traffic regulations, addressing anxiety and enhancing overall intersection performance.
The proliferation of personal mobility devices, such as e-bikes and e-scooters, introduces a new wrinkle into the mix. These devices can move faster than traditional bicycles, posing challenges for signal timing and overall traffic management. This emphasizes the need for adjustments to accommodate the evolving nature of urban mobility. Enhancing the visibility of crosswalks, whether through raised crosswalks or contrasting colors, can make a significant difference in pedestrian safety by increasing driver awareness. Likewise, the synchronization of street lights with traffic signals can enhance visibility and traffic flow in areas with high pedestrian and cyclist activity, creating a more cohesive and user-friendly urban environment.
The use of pedestrian countdown timers is another effective strategy for reducing pedestrian anxiety and encouraging safe crossing behavior. These timers help to manage pedestrian expectations and facilitate more informed decisions during street crossings. The increasing use of real-time traffic and pedestrian data is also significantly changing the optimization landscape. By continuously monitoring usage patterns, engineers can refine signal timings in response to current needs, promoting a more dynamic and responsive urban environment.
Curbside management, including the thoughtful design of areas for ride-share drop-offs, can also play a significant role in easing congestion at intersections. These designs can enhance the pedestrian and cyclist experience in high-traffic areas. Finally, it's important to recognize that engaging the public in traffic signal planning can bring valuable insights into local behaviors and needs. Participatory design approaches can ensure that solutions more closely reflect the unique needs of all urban dwellers, leading to more equitable and effective transportation solutions.
This shift in focus is critical to building a more equitable and sustainable urban transportation system. Addressing the imbalances in current signal optimization practices is essential for promoting safe and efficient movement for all road users. The evolution of technology and the changing landscape of urban mobility necessitate a more nuanced and adaptive approach to traffic signal design.
7 Critical Engineering Challenges in Writing Active Transportation Plans for Urban Areas - Stormwater Management Integration Along Active Transportation Corridors
Integrating stormwater management into the design of active transportation corridors is increasingly important in urban areas. As cities grow and weather patterns change, traditional stormwater systems are often overwhelmed during heavy rain events. This has led to a greater focus on finding ways to manage stormwater more effectively, particularly along paths frequently used by walkers and cyclists.
Solutions like Green Stormwater Infrastructure (GSI) show promise for incorporating natural processes into urban landscapes, improving flood mitigation while potentially enhancing the quality of transportation routes. This approach requires collaboration between engineers, urban planners, and environmental experts to ensure that stormwater management and active transportation plans work together seamlessly. However, achieving this integration isn't straightforward. Factors like aging infrastructure, environmental concerns, and the complexities of integrating GSI into existing urban structures present significant obstacles.
If these challenges aren't addressed effectively, the benefits of active transportation corridors could be undermined by poor stormwater management. A more holistic perspective that considers both transportation and ecological impacts is needed to create truly integrated and sustainable active transportation networks in urban areas. This requires a move away from viewing stormwater solely as a problem to be addressed through engineered solutions, and instead treating it as a critical element in a well-designed urban environment.
Incorporating stormwater management into active transportation corridors offers a promising way to mitigate urban flooding and improve water quality. Using permeable pavement and bioswales allows rainwater to soak into the ground instead of flowing rapidly into storm drains, which often can't handle the increased flow during heavy downpours. It's particularly important to focus on the initial rush of water after a rainfall, the "first flush," as it carries a high concentration of pollutants.
The design of these corridors themselves impacts local water flow patterns. Ideally, we'd want them to mimic natural water cycles to prevent erosion and the movement of sediment downstream. Subtle changes in the road surface, like creating small dips or variations in height (microtopography), can significantly enhance the ability of the ground to absorb rainwater. Incorporating elements like rain gardens and green roofs not only improves stormwater management but can also enhance urban aesthetics.
However, this approach requires flexibility. Urbanization patterns change, and water quality and quantity fluctuate, making it important for designs to be adaptable to changing conditions. The materials we use are also critical—porous asphalt can make a big difference in water infiltration rates compared to traditional asphalt. We have to factor in the specific climate of the region. Areas with intense rainfall might need more robust drainage systems to prevent street damage, while drier areas might emphasize retaining water.
Effective planning for stormwater management in these corridors demands close collaboration between engineers specializing in both transportation and hydraulics. This ensures that the designs meet both the transportation needs and the specific water flow characteristics of the location. While there's an initial investment needed to create these systems, they provide long-term economic benefits by reducing the need for costly repairs and extending the life of roadways. This can lead to substantial savings for municipalities over time, which is an increasingly important consideration with aging infrastructure and growing populations.
However, we have to be careful not to oversimplify the complexity of designing for both transportation and stormwater. It’s easy to imagine an ‘ideal’ system, but the real world presents a lot of challenges. This is where a strong research perspective is important. As we go forward, research should always be exploring better ways to understand and address the unique challenges of each setting, especially given the growing uncertainty in future precipitation patterns due to climate change.
7 Critical Engineering Challenges in Writing Active Transportation Plans for Urban Areas - Right of Way Allocation Standards for Complete Streets Implementation
Implementing "Complete Streets" necessitates a fundamental shift in how we design urban roadways. The goal is to create a transportation network that serves everyone, not just vehicles. This requires establishing clear standards for how we allocate space within the public right-of-way, putting a greater emphasis on the needs of pedestrians and cyclists. A key element of this is defining a modal hierarchy, where safety and accessibility become the primary concerns, especially for those most vulnerable on the road.
While some efforts have been made to implement these standards, progress has been uneven and hasn't fully integrated into practice, particularly at the national and state level. Implementing them effectively in urban environments continues to present a significant hurdle, demanding ongoing collaboration between disciplines, and the development of innovative design approaches.
It is crucial to recognize the value of continuous and dedicated infrastructure for active transportation, such as well-maintained sidewalks and clearly defined bike lanes. These dedicated facilities help create the safe and connected environments that are vital to the success of the "Complete Streets" concept. The ultimate aim of these right-of-way allocation standards is to create a transportation system that promotes fairness and equity, benefiting everyone and contributing to a more sustainable urban future. However, achieving this vision will require persistent attention and a willingness to overcome the challenges that stand in the way.
Complete Streets, a concept aiming for safe and accessible transportation for all users, requires careful consideration of right-of-way (ROW) allocation. While there's been progress in allocating ROW for Complete Streets over the last decade, there's still much to be done in solidifying practices and establishing national standards.
The width and allocation of ROW vary significantly across the world. For example, shared-use paths can range from 10 feet to over 20 feet, highlighting how location and context heavily influence these decisions. This process needs the collaboration of transportation engineers, urban designers, public health experts, and the communities impacted, demonstrating the broader societal aspects of ROW beyond pure engineering.
Research suggests that increasing pedestrian space, even a few feet, can significantly reduce injury rates. So, understanding how changes in ROW affect safety is vital. Additionally, the design of ROW must account for turning radii of vehicles to prevent accidents involving larger vehicles and cyclists or pedestrians. Different legal frameworks influence how cities manage ROW, creating a complex landscape for engineers when trying to implement changes.
Equity needs to be a focal point in ROW allocation as underserved communities may have limited access to safe transportation options. Designing for fairness, such as including more shared streets in these neighborhoods, can promote more equitable mobility. We also need to keep adapting as the urban transportation environment is dynamic. With new mobility options like e-scooters and bikeshare systems, ROW allocation standards need to change to accommodate shifting traffic patterns.
Public involvement is crucial to successful Complete Streets implementation. When communities participate, the outcome tends to better meet the local needs, resulting in higher utilization. Even the materials used for road surfaces impact how effectively ROW can be allocated. For instance, porous asphalt offers both better stormwater management and improved traction for cyclists.
We're seeing more and more use of technologies like data analytics and simulations in ROW planning. These tools allow engineers to better predict traffic flow and user behavior, resulting in more informed decisions when designing Complete Streets. While progress has been made, it's clear that the journey toward effective ROW allocation for Complete Streets is ongoing and presents many unique challenges in various contexts.
7 Critical Engineering Challenges in Writing Active Transportation Plans for Urban Areas - Load Bearing Requirements for Multi Modal Bridge Infrastructure
Multimodal bridge infrastructure, designed to accommodate various transportation modes, presents significant challenges regarding load-bearing capacity. Existing bridges, often built with older standards, might not be able to handle the increased loads of contemporary transportation needs, including heavier vehicles and the growing popularity of cycling and walking. Determining the precise load-carrying capacity of these structures is a key engineering challenge, particularly as we see shifts in traffic patterns and the adoption of new technologies, such as specialized bridge bearings that can better manage movement and weight distribution.
Effectively planning and building new bridge infrastructure requires updated load models that account for the specific stresses imposed by each user group. Whether it's a bus, a delivery truck, or a pedestrian, engineers must ensure the bridge elements are strong enough to safely bear these various weights. Furthermore, bridge design must adhere to both serviceability and ultimate limit state criteria. Serviceability standards ensure the bridge remains usable and performs adequately under typical traffic loads. The ultimate limit state design accounts for the worst-case scenarios to ensure the bridge's structural integrity and the safety of all users. Balancing these various demands—structural integrity, usability, and safety—within the complex environment of an urban area is a critical engineering challenge that requires innovative solutions.
1. **Balancing Diverse Loads**: Designing bridges for multiple uses, like cars, bikes, and pedestrians, requires careful consideration of a wide range of load types. Engineers grapple with how these different loads interact, often using complex computer models (like finite element analysis) to understand how the bridge might respond to various scenarios.
2. **Adapting to Changing Loads**: Some new bridge designs include systems that can adjust to the constantly changing load conditions. These adaptive load management systems (ALMS) are a fascinating development, allowing the bridge structure itself to react in real-time. The hope is that ALMS can enhance safety and possibly even make the best use of materials.
3. **The Challenge of Fatigue**: Bridges that handle a mix of heavy vehicles and lighter users, like cyclists, face a particular challenge: fatigue loading. This is when repetitive smaller loads, like many cyclists, can cause wear and tear over time. Engineers need to recognize this unique aspect of multi-modal bridge design and factor it into the overall design.
4. **Predicting the Future**: The lifespan of a bridge depends on the loads it's subjected to. A bridge mainly used by heavy trucks will have a different lifespan than one used by many pedestrians and cyclists. So, when designing a multi-modal bridge, it's crucial to carefully consider these different usage patterns and how they impact the bridge's expected life.
5. **Withstanding Earthquakes**: In areas prone to earthquakes, load-bearing requirements become even more vital. Engineers rely on sophisticated simulation methods to understand how a bridge might respond to these extreme events, aiming for structures that can withstand seismic loads.
6. **Smart Material Choices**: The type of materials used in a bridge can have a huge effect on its load-bearing capacity and overall behavior. For instance, new composite materials are stronger and lighter than traditional materials, opening up the possibilities for more innovative designs.
7. **Understanding Dynamic Loads**: The loads on a bridge aren't always static; they change as vehicles move. The speed, acceleration, and potential impacts of vehicles all contribute to dynamic loads. Engineers have to carefully incorporate these dynamic aspects into their calculations to ensure safety.
8. **Sharing the Load**: Having multiple modes of transport on a bridge can lead to complex load distribution challenges. Understanding how the load is spread across the structure is vital, and this often requires detailed mathematical models to predict how the bridge will respond under different usage patterns.
9. **Thinking Long-Term**: Taking a life-cycle assessment (LCA) approach to bridge design is becoming more common. This means considering how loads and usage might change over time, helping engineers anticipate future needs and design more adaptable bridges.
10. **Navigating a Regulatory Maze**: The rules governing the design of bridges can vary widely between locations. Engineers need to ensure their designs follow all relevant codes and standards, while also fulfilling the unique requirements of multi-modal bridge designs, which can make the process a bit more complex.
More Posts from specswriter.com: